A dual-mode wearable sensor with coupled ion and pressure sensing
Abstract
Simultaneous monitoring of the body’s biochemical and biophysical signals via wearable devices can provide a comprehensive assessment of an individual’s health state. However, current multifunctional sensors for synchronous biochemical and biophysical sensing rely on discrete sensing units, posing a limitation in increased complexity in device assembly, signal processing, and system integration. In this study, we report a dual-mode and self-powered wearable sensor with ion and pressure-sensing capabilities by interfacing a hydrogel film with a solid ion-selective electrode. The hydrogel film can not only collect natural sweat from the skin but also offer a piezoionic response to pressure. We show that wrist pulse-induced pressure response can be incorporated into the noise of the response to sweat sodium ions, allowing for the simultaneous measurement of heart rate and sweat electrolytes. This work provides an example of simplifying the development of wearable multimode sensors through the rational design of classic electrochemical sensors.
Keywords
INTRODUCTION
Skin-mounted wearable sensors are integrated analytical devices that can monitor various physiological signals in a real-time and continuous way[1-5]. Their point of care feature, along with non-invasive or minimally invasive operations, provides a promising pathway to user-friendly healthcare and diagnostics[6-8]. Wearable sensors can be classified into physical and biochemical types based on target physiological signals. Specifically, wearable physical sensors are designed to monitor biophysical signals such as body motions, blood pressure, heart rate, or body temperature[9-13], while biochemical sensors focus on the analysis of biomarkers in body fluids to indicate the health state at the molecule level[14-17]. The comprehensive evaluation of the body state for precious health management and diagnosis calls for next-generation hybrid wearables capable of physical and biochemical sensing concurrently[18,19]. A straightforward way to achieve this goal is integrating various sensors with different sensing mechanisms into a single device[20-22]. However, this add-on design inevitably results in increased complexity in fabrication, signal processing, and device integration.
Among various biofluids, sweat may be the best choice for wearable sensing to indicate the dynamic body state at the molecular level[23]. This is because sweat contains a wealth of biochemical information that is composed of various metabolites (glucose, lactate), electrolytes (sodium, chloride, potassium), proteins, hormones, and peptides[24]. Moreover, compared to other biofluids such as tear and saliva, sweat can be easily accessed in a non-invasive and on-demand manner and readily analyzed using skin-interfaced electronic devices. Among various sweat electrolyte ions, the level of sodium ions (Na+) can reflect the body’s electrolyte balance, osmolality regulation, and acid-base equilibrium, which is also a biomarker for the diagnosis of cystic fibrosis[25-27]. Among the biophysical signals, the pulse caused by the periodic flow of blood can reflect the health state of the heart, and pulse monitoring has wide applications in the diagnosis of cardiovascular diseases, physical fitness, and health management[28]. Simultaneous detection of these two basic yet important physiological signals may give a full picture of the health state of an individual. However, this can only be done using two sensors with existing strategies.
Herein, we show that a single wearable sensing unit can detect sweat Na+ concentration and heart rate simultaneously. This was achieved by introducing a hydrogel interface to a solid Na+ selective electrode. In previous reports, the introduction of a hydrogel film between skin and electrochemical sensing electrodes has proven to be an efficient way to continuously collect and analyze natural sweat[16,29]. Thus, the hydrogel interface is quite attractive to eliminate the use of chemical, exercise, or thermal stimuli for sweat induction and may offer unique insight into body physiology at the rest state[30]. In this work, considering hydrogels also have the piezoionic response to pressure[31-33], we show that the wrist pulse-induced potential signal with a periodic pattern can also be monitored during the sensing of sweat Na+. Thus, the pulse and sweat Na+ concentration can be easily analyzed from the single potential response. We believe this dual-mode sensor has the potential to pave the way for the next generation of multifunctional wearable sensors for synchronous biophysical and biochemical sensing.
EXPERIMENTAL
Chemicals and materials
Selectophore grade sodium ionophore X, 3,4-ethylene-dioxythiophene (EDOT), poly (sodium 4-styrene sulfonate) (NaPSS), sodium tetrakis [3,5-bis(trifluoromethyl)phenyl] borate (Na-TFPB), polyvinyl chloride (PVC), bis(2-ethylhexyl) sebacate (DOS), acrylamide (AM), N, N’-Methylenebisacrylamide (Bis), poly (ethylene glycol) diacrylate (PEGDA), and (2-hydroxy-2-methylpropiophenone) (HMPP) were obtained from Sigma-Aldrich. Polyvinyl butyral (PVB) and sodium chloride (NaCl) were obtained from Aladdin (Shanghai, China). Tetrahydrofuran and methanol were obtained from Macklin (Shanghai, China). A CHI 660D electrochemical analyzer (CH Instruments) was used to carry out electrochemical measurements. A commercial blood pressure and pulse meter (YE 660D, Yuyue Medical Co., Ltd., China) was used for the pulse test.
Preparation of the hydrogel
The p(AM-co-PEGDA) hydrogel was prepared by dissolving 10 wt% AM, 0.2 wt% Bis, 1 wt% PEGDA, and
Preparation of the Na+ selective electrode
Graphite ink (LOCTITE EDAG423SS E&C) was screen-printed on the poly (ethylene glycol) terephthalate (PET) film, followed by drying at 90 °C for 30 min. The reference electrodes were printed with silver/silver chloride ink (LOCTITEEDAG 6037SS E&C) followed by drying at 80 °C for 30 min [Supplementary Figure 2][34]. Then, the poly(3,4-ethylene-dioxythiophene) PEDOT:PSS was deposited on the working electrodes as an ion-electron transducer through the galvanostatic electrochemical polymerization in a solution containing 100 mM NaPSS and 10 mM EDOT. A constant current of 35 μA was applied, and the depositing time was 285 s. We prepared the Na+ selective membrane solution by dissolving 1.0 wt% Na ionophore X, 0.55 wt% Na-TFPB, 33 wt% PVC, and 65.45 wt% DOS in tetrahydrofuran and a PVB-based coating solution by dissolving 79 mg PVB and 50 mg NaCl in 1.0 mL methanol. Finally, 2.0 μL of Na+ selective membrane solution and 2.0 μL of PVB solution were dropped on the working electrodes and reference electrodes, respectively, followed by overnight drying at room temperature [Supplementary Figure 3].
On-body test
To perform the sweat rate test, a piece of filter paper (Cytiva, 0.5 cm × 1 cm) was attached to the wrist to absorb sweat for an hour. During this process, the paper was weighed every ten minutes. Before each test, the sweat collection area at the wrist was cleaned with 75% alcohol and deionized water, followed by removing the water using absorbent cotton. Then, the sensor was positioned on the wrist and covered by a waterproof adhesive layer to prevent water evaporation. Before the on-body test, the hydrogel was immersed in 1 mM NaCl solution. All experiments were conducted in strict compliance with the relevant laws and with the approval of the Scientific Ethical Committee of Southeast University. Three healthy volunteers were recruited to participate in the on-body tests.
RESULTS AND DISCUSSION
Overview of the dual-mode sensor
Existing strategies for wearable sweat Na+ detection include optical techniques, e.g., fluorescence or colorimetric analysis[35,36], and electrochemical methods using potentiometric ion selective electrodes (ISE)[37] or organic electrochemical transistors[38]. Among these sensing methods, ISE-based Na+ sensors are particularly attractive due to their simple electrode and circuit design, low cost, and ability for continuous sensing[34].
Here, we designed the wearable dual-mode sensor with ion and pressure sensing functions by interfacing a hydrogel film to a solid Na+ selective electrode [Supplementary Figure 4], which was attached to the wrist to monitor the sweat Na+ and pulse simultaneously [Figure 1A]. In our design, the hydrogel film can be used not only for sweat collection but also for pressure sensing by utilizing the piezoionic effect of the hydrogel. The piezoionic electromechanical response of hydrogel is due to the difference in mobilities of cations and anions in the hydrogel that generates a streaming potential when pressure is applied[32] [Figure 1B]. Although the responses to sweat Na+ and pressure are all based on the potential variation of the sensor, we can differentiate the wrist pulse from the Na+ response curve due to its periodic pattern. Moreover, the wrist pulse-induced pressure response is at the noise level of the potential signal, and thus, we can synchronously obtain the sweat Na+ level and heart rate [Figure 1C]. To our knowledge, this is the first report using a single wearable electrochemical sensor to achieve simultaneous chemical and biophysical sensing. In addition to dual-mode sensing, this sensor is also operated in a self-powered way without using complicated peripheral circuits for signal readout.
Figure 1. Schematic illustration of the dual-mode wearable sensor. (A) Schematic illustration showing the dual-mode sensor working as a wearable device; (B) Structure and working mechanism of the dual-mode sensor; (C) (i-iii) Schematic illustration of the dual-mode sensor detecting (i) the sweat Na+, (ii) periodic pressure, and (iii) the two physiological signals simultaneously.
Detection of the Na+ in the hydrogel
We utilized the biocompatible p(AM-co-PEGDA) hydrogel film to interface the skin and sensing electrodes for collecting natural sweat and pulse monitoring. Cross-sectional SEM image indicated the porous architecture of hydrogel with a mean pore size of 13.7 μm [Supplementary Figure 5]. In addition, the pore sizes of the hydrogel equilibrated in NaCl solution with varied concentrations (0, 1, 5, and 10 mM) changed slightly, and the mean pore size ranged from 13.5 to 15.3 μm [Supplementary Figure 6]. The in vitro cytotoxicity assay was performed with NIH 3T3 cells using a standard Cell Counting Kit-8 (CCK8) assay, and the results indicated the good biocompatibility of the p(AM-co-PEGDA) hydrogel [Supplementary Figure 7]. Furthermore, the on-skin test indicated there was no irritation reaction, such as erythema and edema, after wearing for 2 h [Supplementary Figure 8]. In addition, the water content of the p(AM-co-PEGDA) hydrogel remained nearly unchanged after ten days, indicating its good stability [Supplementary Figure 9].
To characterize the Na+ selective electrode’s response to the Na+ in the hydrogel, we immersed the hydrogels in NaCl solution with different concentrations (4, 8, 16, 32, and 64 mM) and then attached the hydrogels to the electrode surface. The open-circuit potential monotonously increased as the Na+ concentration in the hydrogel increased [Figure 2A]. Figure 2B shows the potential value plotted against the logarithm of Na+ concentration with a correlation coefficient of 0.999, indicating that the quantitative analysis of Na+ in the hydrogel can be achieved. It is noted that these results were consistent with those obtained by directly detecting the corresponding NaCl solutions using the Na+ selective electrode [Supplementary Figure 10]. In addition, the Na+ selective electrode exhibited not only selectivity of Na+ but also good anti-interference to other cations [Supplementary Figure 11].
Figure 2. Detection of the Na+ in the hydrogel. (A) Open-circuit potential in response to hydrogels containing NaCl solutions with different concentrations; (B) Plots of the potential value as a function of the logarithm of the Na+ concentration in the hydrogel; (C) Potential change after adding NaCl solution to the hydrogel.
To simulate the process of the hydrogel collecting the sweat, we dropped 2.0 μL of 100 mM NaCl solution on the hydrogel. The potential increased once the NaCl solution was added to the hydrogel [Figure 2C]. Then, the potential stabilized after ~150 s due to the diffusion and equilibrium of Na+ in the hydrogel film. The measured Na+ concentration is 8.36 ± 1.1 mM (n = 5), which is close to the theoretical value of 9.09 mM. Furthermore, we attached the sensor to the wrist of a volunteer to detect the volunteer’s sweat Na+ concentration. Based on the measured sweat rate [Supplementary Figure 12], the volume of sweat absorbed by the hydrogel can be calculated. We then tracked the sweat Na+ concentration of the volunteer undergoing different activities, including exercise and drinking water [Supplementary Figure 13]. The volunteer’s sweat Na+ concentration was 8.0 mM before the exercise. After 30 min of exercise, the sweat Na+ concentration increased to 9.6 mM. Then, the sweat Na+ concentrations returned to 7.5 mM due to the water intake and regulation of hydration. The measured sweat Na+ was slightly below the typical physiological range of sweat Na+ (10-100 mM)[39]. This may be due to the low sweat rate since the test was conducted when the volunteer was at the rest state, and previous reports have shown a positive correlation between sweat Na+ concentration and sweat rate[40].
Pressure sensing of the dual-mode sensor
In addition to the sweat collection, the hydrogel containing free Na+ and Cl- can also be used as a pressure sensor by generating potential in response to mechanical pressure stimuli. Due to the different mobility of the Na+ and Cl-, an ion gradient is established, and a voltage signal is generated in the hydrogel under pressure, namely, the piezoionic effect of the hydrogel[32]. Herein, we used the Na+ selective electrode to detect the generated potential of the hydrogel by interfacing the hydrogel on the electrodes. When we exert pressure on the working electrode, a decrease in potential is detected since the diffusion rate of Cl- is lower than that of Na+ [Figure 3A].
Figure 3. Pressure sensing performance of the dual-mode sensor. (A) Schematic diagram of the piezoionic mechanism of the hydrogel; (B) Cyclic pressure/release test for ten cycles; (C) Generated potential as a function of the applied pressure; (D) On-body pulse detection by fixing the pressure sensor at the wrist; (E) Generated potential as a function of the NaCl concentration in the hydrogel under the pressure of 15 kPa; (F) Open-circuit potential change when applying periodic pressure of 15 kPa with a frequency of 20 and dropping 2.0 μL NaCl solution (10 mM) on the hydrogel containing NaCl solution (1 mM).
To show the piezoionic effect of the hydrogel, a cyclic pressure/release test at a pressure of 15 kPa over ten cycles was conducted, and the potential response is shown in Figure 3B. The pressure induced a decrease in potential, and the potential could rapidly return to its original level in a short time (< 0.5 s) once the pressure was removed. We also applied different pressures on the hydrogel containing 1 mM NaCl solution. The potential change linearly increased with rising pressure (R2 = 0.997) and exhibited a sensitivity of
Furthermore, we applied periodic pressure with a frequency of 20 and added 2.0 μL of 10 mM NaCl solution to the hydrogel with 1 mM NaCl solution, simulating the simultaneous detection of the change in Na+ concentration and pressure [Figure 3F]. Before adding the NaCl solution, the stable potential baseline of
On-body real-time monitoring of sweat Na+ and pulse
The dual mode sensor was used to monitor sweat Na+ and pulse simultaneously by attaching it to the wrist of a female volunteer aged 25 [Figure 4]. Before the test, a waterproof film was added between the skin and hydrogel to prevent the hydrogel from absorbing sweat; thus, the potential baseline remained stable, and the periodic fluctuation was caused by the pulse. Upon removing the waterproof film, the potential baseline gradually increased with time as the hydrogel collected the Na+ from sweat. After five minutes, the waterproof film was added again. As a result, the potential baseline stabilized to -43 mV while the pulse potential signal remained. The rising amplitude of the potential baseline from -47 to -43 mV meant that the Na+ concentration increased from 1 to 1.10 mM, and the sweat Na+ concentration was 6.99 mM. In addition, the pulse information of 89 beats/min can be read out from the number of peaks. Both the Na+ concentration and pulse are in agreement with the results measured separately, demonstrating the practicability of this wearable sensor for the simultaneous detection of the physical and biochemical signals. We also demonstrated the on-body tests on the other two volunteers, and the concurrent pulse monitoring and sweat Na+ sensing can be achieved for each volunteer [Supplementary Figure 16].
CONCLUSIONS
In summary, we developed a dual-mode sensor capable of the simultaneous detection of both physical and biochemical signals by integrating a hydrogel with a Na+ selective electrode. The solid-state Na+ selective electrode was endowed with pressure-sensing capability based on the piezoionic response. In addition, the hydrogel also works as a flexible skin interface for sampling sweat. Thus, we can simultaneously detect the mechanical pressure and Na+ concentration using a single sensor. We also demonstrated its application as a wearable device for the simultaneous detection of sweat Na+ and pulse. We believe that this integrated dual-mode sensor will pave the avenue for the next-generation multifunctional wearable sensors for applications in healthcare.
DECLARATIONS
Authors’ contributions
Conceptualization, methodology, and writing-original draft: Ma B
Methodology and data curation: Huang K
Writing-original draft: Chen G
Investigation: Tian Y, Jiang N
Resources: Zhao C
Writing-review and editing, and funding acquisition: Liu H
Availability of data and materials
Not applicable.
Financial support and sponsorship
The authors gratefully acknowledge the financial support from the Natural Science Foundation of Jiangsu Province (BK20220859), the Key Research and Development Program of Jiangsu Province (BE2021700), the Science and Technology Development Program of Suzhou (SYG202117), the Jiangsu Funding Program for Excellent Postdoctoral Talent, and the China Postdoctoral Science Foundation (2022M710667).
Conflicts of interest
All authors declared that there are no conflicts of interest.
Ethical approval and consent to participate
Not applicable.
Consent for publication
Not applicable.
Copyright
© The Author(s) 2024.
Supplementary Materials
REFERENCES
1. Yu Y, Nyein HYY, Gao W, Javey A. Flexible electrochemical bioelectronics: the rise of in situ bioanalysis. Adv Mater 2020;32:1902083.
2. Ates HC, Nguyen PQ, Gonzalez-Macia L, et al. End-to-end design of wearable sensors. Nat Rev Mater 2022;7:887-907.
4. Singh SU, Chatterjee S, Lone SA, et al. Advanced wearable biosensors for the detection of body fluids and exhaled breath by graphene. Mikrochim Acta 2022;189:236.
5. Shen J, Li B, Yang Y, et al. Application, challenge and perspective of triboelectric nanogenerator as micro-nano energy and self-powered biosystem. Biosens Bioelectron 2022;216:114595.
6. Su Y, Yang T, Zhao X, et al. A wireless energy transmission enabled wearable active acetone biosensor for non-invasive prediabetes diagnosis. Nano Energy 2020;74:104941.
7. Ji W, Zhu J, Wu W, et al. Wearable sweat biosensors refresh personalized health/medical diagnostics. Research 2021;2021:9757126.
8. Wang Y, Haick H, Guo S, et al. Skin bioelectronics towards long-term, continuous health monitoring. Chem Soc Rev 2022;51:3759-93.
9. Trung TQ, Lee NE. Flexible and stretchable physical sensor integrated platforms for wearable human-activity monitoringand personal healthcare. Adv Mater 2016;28:4338-72.
10. Ma B, Xu C, Chi J, Chen J, Zhao C, Liu H. A versatile approach for direct patterning of liquid metal using magnetic field. Adv Funct Mater 2019;29:1901370.
11. Gao FL, Min P, Gao XZ, et al. Integrated temperature and pressure dual-mode sensors based on elastic PDMS foams decorated with thermoelectric PEDOT:PSS and carbon nanotubes for human energy harvesting and electronic-skin. J Mater Chem A 2022;10:18256-66.
13. Byfield R, Miller M, Miles J, Guidoboni G, Lin J. Towards robust blood pressure estimation from pulse wave velocity measured by photoplethysmography sensors. IEEE Sens J 2022;22:2475-83.
14. Sempionatto JR, Lasalde-Ramírez JA, Mahato K, Wang J, Gao W. Wearable chemical sensors for biomarker discovery in the omics era. Nat Rev Chem 2022;6:899-915.
15. Wang M, Yang Y, Min J, et al. A wearable electrochemical biosensor for the monitoring of metabolites and nutrients. Nat Biomed Eng 2022;6:1225-35.
16. Chen Y, Ma B, Zuo Y, et al. Versatile sweat bioanalysis on demand with hydrogel-programmed wearables. Biosens Bioelectron 2023;235:115412.
17. He T, Wen F, Yang Y, Le X, Liu W, Lee C. Emerging wearable chemical sensors enabling advanced integrated systems toward personalized and preventive medicine. Anal Chem 2023;95:490-514.
18. Gao W, Ota H, Kiriya D, Takei K, Javey A. Flexible electronics toward wearable sensing. Acc Chem Res 2019;52:523-33.
19. Ray TR, Choi J, Bandodkar AJ, et al. Bio-integrated wearable systems: a comprehensive review. Chem Rev 2019;119:5461-533.
20. Gao W, Emaminejad S, Nyein HYY, et al. Fully integrated wearable sensor arrays for multiplexed in situ perspiration analysis. Nature 2016;529:509-14.
21. Nakata S, Arie T, Akita S, Takei K. Wearable, flexible, and multifunctional healthcare device with an ISFET chemical sensor for simultaneous sweat pH and skin temperature monitoring. ACS Sens 2017;2:443-8.
22. Zhang X, Xia Y, Liu Y, Mugo SM, Zhang Q. Integrated wearable sensors for sensing physiological pressure signals and β-hydroxybutyrate in physiological fluids. Anal Chem 2022;94:993-1002.
23. Min J, Tu J, Xu C, et al. Skin-interfaced wearable sweat sensors for precision medicine. Chem Rev 2023;123:5049-138.
25. Kaur G, Kaur N. Estimation of sodium ions using easily engineered organic nanoparticles-based turn-on fluorescent sensor: application in biological and environmental samples. Sens Actuators B Chem 2018;265:134-41.
26. Gonçalves AC, Marson FAL, Mendonça RMH, et al. Chloride and sodium ion concentrations in saliva and sweat as a method to diagnose cystic fibrosis. J Pediatr 2019;95:443-50.
27. Speedy DB, Noakes TD, Schneider C. Exercise-associated hyponatremia: a review. Emerg Med 2001;13:17-27.
28. Meng K, Xiao X, Wei W, et al. Wearable pressure sensors for pulse wave monitoring (Adv. Mater. 21/2022). Adv Mater 2022;34:2270158.
29. Lin PH, Sheu SC, Chen CW, Huang SC, Li BR. Wearable hydrogel patch with noninvasive, electrochemical glucose sensor for natural sweat detection. Talanta 2022;241:123187.
30. Nyein HYY, Bariya M, Tran B, et al. A wearable patch for continuous analysis of thermoregulatory sweat at rest. Nat Commun 2021;12:1823.
31. Wu X, Zhu J, Evans JW, Lu C, Arias AC. A potentiometric electronic skin for thermosensation and mechanosensation. Adv Funct Mater 2021;31:2010824.
32. Dobashi Y, Yao D, Petel Y, et al. Piezoionic mechanoreceptors: force-induced current generation in hydrogels. Science 2022;376:502-7.
33. Li J, Li J, Tang Y, et al. Touchable gustation via a hoffmeister gel iontronic sensor. ACS Nano 2023;17:5129-39.
34. Ma B, Chi J, Xu C, Ni Y, Zhao C, Liu H. Wearable capillary microfluidics for continuous perspiration sensing. Talanta 2020;212:120786.
35. Sekine Y, Kim SB, Zhang Y, et al. A fluorometric skin-interfaced microfluidic device and smartphone imaging module for in situ quantitative analysis of sweat chemistry. Lab Chip 2018;18:2178-86.
36. Choi J, Bandodkar AJ, Reeder JT, et al. Soft, skin-integrated multifunctional microfluidic systems for accurate colorimetric analysis of sweat biomarkers and temperature. ACS Sens 2019;4:379-88.
37. Glennon T, O’quigley C, McCaul M, et al. ‘SWEATCH’: a wearable platform for harvesting and analysing sweat sodium content. Electroanalysis 2016;28:1283-9.
38. Demuru S, Kunnel BP, Briand D. Real-time multi-ion detection in the sweat concentration range enabled by flexible, printed, and microfluidics-integrated organic transistor arrays. Adv Mater Technol 2020;5:2000328.
39. Parrilla M, Ortiz-Gómez I, Cánovas R, Salinas-Castillo A, Cuartero M, Crespo GA. Wearable potentiometric ion patch for on-body electrolyte monitoring in sweat: toward a validation strategy to ensure physiological relevance. Anal Chem 2019;91:8644-51.
Cite This Article
Export citation file: BibTeX | RIS
OAE Style
Ma B, Huang K, Chen G, Tian Y, Jiang N, Zhao C, Liu H. A dual-mode wearable sensor with coupled ion and pressure sensing. Soft Sci 2024;4:8. http://dx.doi.org/10.20517/ss.2023.41
AMA Style
Ma B, Huang K, Chen G, Tian Y, Jiang N, Zhao C, Liu H. A dual-mode wearable sensor with coupled ion and pressure sensing. Soft Science. 2024; 4(1): 8. http://dx.doi.org/10.20517/ss.2023.41
Chicago/Turabian Style
Ma, Biao, Ke Huang, Gangsheng Chen, Yingnan Tian, Nan Jiang, Chao Zhao, Hong Liu. 2024. "A dual-mode wearable sensor with coupled ion and pressure sensing" Soft Science. 4, no.1: 8. http://dx.doi.org/10.20517/ss.2023.41
ACS Style
Ma, B.; Huang K.; Chen G.; Tian Y.; Jiang N.; Zhao C.; Liu H. A dual-mode wearable sensor with coupled ion and pressure sensing. Soft. Sci. 2024, 4, 8. http://dx.doi.org/10.20517/ss.2023.41
About This Article
Special Issue
Copyright
Data & Comments
Data
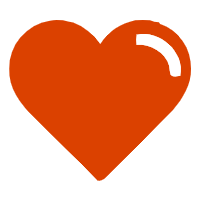

Comments
Comments must be written in English. Spam, offensive content, impersonation, and private information will not be permitted. If any comment is reported and identified as inappropriate content by OAE staff, the comment will be removed without notice. If you have any queries or need any help, please contact us at support@oaepublish.com.