The sweat rate as a digital biomarker in clinical medicine beyond sports science
Abstract
Sweating is an important physiological reaction and a clinical symptom in a variety of diseases. However, it remains underrated in clinical use. Gold standards to measure the sweat rate are neither continuous nor easily or lab-independently applicable. With the emergence of novel wearable devices, using the sweat rate as a digital biomarker shows promise for clinical monitoring and diagnostics. In this Commentary, we discuss the potential and importance of the sweat rate as a digital biomarker in clinical medicine beyond sports science.
Keywords
INTRODUCTION
Sweating is an important physiological reaction to maintain the body’s thermoregulation during exposure to environmental heat stress or during rigorous exertion. This is one of the main reasons why the sweat rate assessment has been increasingly investigated in sports science and occupational health since the emergence of wearable sweat sensing. Reports from diseases such as the “Sudor Anglicus” in the 15th century, alongside common medical knowledge from oncology and infectious diseases, have shown that sweating has not only a thermo-regulatory function but it can be a symptom in clinical medicine as well[1-3]. Therefore, sweat analysis provides valuable information about health, disease, and even age[4,5]. Still, the sweat rate remains underexplored and underrated in clinical medicine. However, with the emergence and continuous advancements of sweat analyzing wearables, sweat rate analysis holds the potential to become a clinically, broadly available digital biomarker. In this Comment, we introduce and discuss the sweat rate as a promising novel digital biomarker to monitor health and disease in clinical medicine beyond sports science.
THE SWEAT RATE PHYSIOLOGY
Basics
The human body houses approximately two to four million sweat glands[6,7]. Eccrine, apocrine, and apoeccrine sweat glands can be distinguished[8,9]. While eccrine sweat glands are responsible for the highest volume of sweat excretion[10], secretion of apocrine and apoeccrine glands can also influence the sweat composition at the skin surface[11]. During sweating, up to 2,426 J of heat per gram of evaporated sweat can be dissipated from the body[12]. Aiming to maintain thermoregulation, sweating is the most efficient way to dissipate heat from the body. Next to heat stress, sweat glands can be stimulated by emotional stress[13], mechanical vibration[14], eating spicy food (gustatory hyperhidrosis)[15], or chemical substances such as carbachol and local current[16].
Neurological control
Many thermosensitive neurons can be found in the preoptic area and the anterior hypothalamus[17]. To keep the body temperature constant, they initiate appropriate responses when detecting changes in body temperature[17]. Augmented local preoptic temperature or a rise in afferent impulses from the cutaneous and spinal thermoreceptors caused by elevated skin temperature can both result in increased sweating[17]. However, increased core body temperature stimulates the sweat rate nine times more efficiently than increased mean skin temperature[18-20].
Body map
Local sweat rates for the mid-front, sides, and mid-later back were found to be significantly higher in males compared to females[21]. In both sexes, the highest sweating was observed along the spine, whereas the sweat rate on the upper arm was lowest. Furthermore, total sweating on the back exceeded the total sweating of the chest.
For older males, gross sweat loss and regional sweat rates were significantly lower compared to the young[22]. During rest, significantly lower regional sweat rates at almost all body regions were observed, whereas, during exercise, a significant difference was found for the hands, legs, ankles, and feet[22].
Influencing variables
Environmental factors such as ambient temperature, air velocity, and radiant load, along with factors such as clothing and the level of physical activity, influence the sweating rate [Figure 1][23].
Figure 1. Factors influencing the sweat rate. Non-comprehensive overview of environmental/physiological and pathophysiological factors influencing the sweat rate, demonstrating the sweat rate as a digital biomarker going beyond sports science and occupational health. Created with BioRender.com.
Heat acclimatization of five to eight days results in thermoregulatory adaptions such as increased sweat rate and earlier onset of sweating[24]. However, depending on humid or dry heat exposure, the adaptation of the eccrine sweat gland differs: the sweat rate in a hot-humid environment is greater than in a hot-dry environment[24]. In healthy unacclimatized men, a sweating capacity of maximally 1.5 liters per hour has been reported, whereas, in highly trained acclimatized soldiers, a sweat rate of two to three liters per hour was reached[25]. Sweat rates of one liter per hour occur frequently depending on factors like the environment or the intensity of exertion; however, sweat rates can vary considerably[26]. Not only heat acclimatization but also training can lead to an increase of sweat rates of 200 to 300 mL per hour[26].
WEARABLE SWEAT RATE ANALYSIS
Diagnostic instruments that rely on sweat samples collected using absorbent pads yield a wealth of information related to physiological status and athletic performance[27,28]. The protocols and the benchtop systems required for this purpose are, however, incompatible with real-time monitoring in the field. This is due to the bulk and expense of the hardware and the time and effort required for sample collection and preparation. Recent advances in flexible, hybrid electronics, soft microfluidics, and electrochemical sensors serve as foundations for emerging classes of skin-mounted systems for measuring the properties of sweat, each with features that overcome key limitations of conventional technologies[29-33].
The measurement of the sweat rate in skin-mounted systems occurs through microfluidic devices that capture the sweat directly from the glands [Figure 2]. The pressure that drives fluid flow arises from the action of the sweat glands themselves, assisted by capillary effects in the microchannels. The microfluidic system usually consists of a thin polymer layer [usually polydimethylsiloxane (PDMS)] embossed with appropriate relief geometry with a top-capping polymer layer [i.e., PDMS and polyethylene terephthalate (PET)] that serves as a seal[33]. The resulting overall thickness (usually smaller than 1 mm) and the addition of adhesive films enable intimate contact with the epidermis. Sweat rates strongly depend on the body location and the intensity of the exercise and may range from 39 to 614 g·h-1·m-2[22]. Therefore, microfluidic devices are typically designed to accommodate tens of uL. The inlet opening of a few mm in diameter enables sweat collection of multiple glands, sometimes with the help of hydrophilic fillers for rapid sweat uptake[34]. The pressure that drives fluid flow arises from the action of the sweat glands themselves, assisted by capillary effects in the microchannels and the materials embedded within them. The measurement of the sweat rate can be implemented through various strategies that can be roughly categorized by transduction methods: (i) optical/visual; (ii) electrical (impedance, resistive). The former consists of fully passive devices (do not need a battery) in which the volume change is estimated visually or with the help of a camera[35-37]. More sophisticated designs offer quantitative information. They usually see the embedding of conductive traces or pads into the microfluidic channels. The flow of sweat induces changes in the electrical impedance. Initial designs suffered from the interdependence of the impedance on the volume and ionic concentration of the sweat, making the estimation of the rate difficult[34,38,39]. More recent designs have overcome this problem by (i) introducing differential measurements through two microfluid systems on the same patch (one for the ionic concentration and one for the rate)[40] and by (ii) patterning an array of pads along the channel to register discrete/digital changes of the impedance that enable time-volume synchronization independently from the ionic concentration[41]. It is worth mentioning that both methods require AC measurements to avoid the accumulation of ionic charge in the channels and the fouling of the impedance readout. This requirement complicates the circuit design of the wearable patch. While the previously mentioned devices rely on the indirect estimation of the rate via the measurement of the sweat volume and the passage time between some markers, another recent solution relies on the implementation of a flowmeter. This method involves reading the electrical resistance of two thermistors positioned on top of the microchannel and spaced out by a heater[42]. The flow of the sweat establishes a temperature change between the two thermistors whose dynamic response correlates with the flow rate. The simplicity of this strategy requires design optimization and intermittent operation to not incur high power consumption due to the heater. It is worth mentioning that all devices listed above are for single use: once the microfluidic is filled up, the device must be replaced by an empty one. Therefore, modular multi-layer designs are exploited to reuse the expensive layer that contains the electronic components and to dispose of the microfluidic layer that can be produced with biodegradable polymers[43]. An overview of typical specifications of wearable sweat rate sensors and a list of reported designs can be found in Table 1. Another major challenge deals with the production and collection of sweat that may be intermittent. Passive and active approaches rely on physical exercises and electro-/chemical stimulation, respectively. Iontophoresis is a widely used method of active sweat induction, allowing the acquisition of sweat samples while the body is sedentary. A current is generated under the skin surface by applying a voltage between the iontophoretic electrodes, allowing the agonist (e.g., pilocarpine molecules) to be delivered to the sweat gland at the anode and stimulating the secretion of sweat[33]. Such an approach has proved to be effective for the monitoring of chloride[44], ethanol[45], c-reactive protein[46], hormones[47], and glucose[44,48]. Other more innovative methods rely on optical infra-red imaging combined with skin temperature and environmental conditions to assess the activity of multiple sweat glands[49] or on the use of sweat-responsive covalent organic films for sweat pores analysis[50]. Currently, we register a few commercial devices that aim to track sweat rates and composition. One is KuduSmart (https://kudusmart.com/), and the other one is the GX patch (https://www.gatorade.com/equipment/gx-sweat-patch/gx-sweat-patch).
Figure 2. Overview of the main proposed approaches to measuring the sweat rate using wearable soft microfluidic sensors.
Typical specifications of wearable sweat rate sensors
Device | Materials and design of µ-fluidics | Sweat rate measurement | Ref. |
1 | A coiled tubing kept in position by a round plastic frame | Sensor: water-responsive chromogenic reagent Read-out: external camera for time-stamped picture Pros/Cons: simple implementation/not thin film so rather bulky, the read-out relies on the post-processing of the picture taken from an external camera (semi-quantitative) | [36] |
2 | A bottom PDMS layer (thickness, 500 μm) embossed with appropriate relief geometry (uniform depth, 300 μm) and with a top-capping layer of PDMS that serves as a seal (thickness, 200 μm) | Sensor: water-responsive chromogenic reagent Read-out: picture taken from an external camera launched by near field communication chip for time Pros/Cons: simple design/ the read-out relies on the post-processing of the picture taken from an external camera (semi-quantitative) | [35] |
3 | Two main parts: (i) a microfluidic; and (ii) an electrical sensing component. The microfluidic channel is prepared with PDMS and is covalently bonded to PET containing sensing electrodes layer, via O2 plasma etching and silanization. In some designs, the collection well is filled with a patterned SU8 filler coated with a thin saturated hydrogel layer that contacts skin for sweat uptake | Sensor: capacitive (analog/continuous). The sweat rate sensor contains two parallel Cr/Au spirals that are aligned with the microfluidic channel. Sweat rate is quantified by the change of impedance Read-out: external PCB for signal processing and communication via bluetooth Pros/Cons: quantitative read-out/the read-out signal depends also on the changing ionic concentration of the sweat | [34,38] |
4 | Design similar to the one of device 3 but with the impedimetric sweat rate sensor formed by two electrodes with interdigitated fingers over which the serpentine channel repeatedly passes | Sensor: capacitive (digital/discrete). The multi electrodes design results in discrete/digital changes of the impedance that enable time-volume synchronization independently form the ionic concentration Read-out: external PCB for signal processing and communication via bluetooth Pros/Cons: quantitative read-out not depending on the ionic sweat concentration/ rather complex implementation (multiple electrodes) | [41] |
5 | The device is formed by three main layers: (i) an adhesive layer to strengthen the contact with the skin; (ii) a PDMS layer with microfluidics and electrodes for sensing; (iii) flexible PCB to connect electronics and communication chips | Sensor: the sensing mechanism relies on the measurement of the resistance between metal pads patterned onto the wall of the microfluidic channels. Two separate channels allow to solved the interdependence of the resistance on rate and electrical conductivity of the sweat. AC modulation is implemented to avoid the formation of electronic double layer that may foul the reading Read-out: flexible PCB for signal processing and communication via NFC Pros/Cons: quantitative read-out not depending on the ionic sweat concentration/rather complex implementation (two separate channels) | [40] |
6 | Design consists of (i) an adhesive layer; (ii) a PDMS microchannel; (iii) a PDMS PCB that connects two thermistors and a heater; and (iv) finally a PDMS cover. The design is simpler than the one of device 3 and 5 since it is rely on the direct measurement of the speed of the sweat flow rather than of the volume. The modular assembly facilitate the re-use of the PCB with with the disposable layers connected via magnets to the flexible PCB | Sensor: the device implements a flowmeter by reading the temperature difference between two thermistors. A heater positioned midway between the thermistors set their temperature which is identical before the flow of the sweat and that is different after. The difference of temperature yields a change of the resistance in the two thermistors Read-out: flexible PCB for signal processing and communication via BLE Pros/Cons: elegant solution implementing a micro-thin film flow-meter/sensitive to stretching because the distance between the two thermistors changes, the heater could be power hungry if not properly biased | [42] |
SWEATING - A SYMPTOM IN CLINICAL MEDICINE
Sweating is an unspecific but very common symptom in clinical medicine. Currently, the quantification of the sweat rate can only be conducted during clinical examination in this field. There are several qualitative measures (the Clinical Opioid Withdrawal Scale and the descriptive observation by the examinator) and quantitative measures (gravimetrical analysis using technical absorbents and determining changes in the body weight) that can be employed[51-53]. An easy, continuous, and straightforward measurement of the sweat rate during everyday life is not yet available. Therefore, the full clinical potential of sweating as a symptom and an indicator of health and diseases has only been vaguely exploited until recently.
Clinical sweat terminology
To objectively assess the symptom of “sweating”, the sweat rate must be determined. It can be distinguished between local and whole body sweat rates. The local sweat rate refers to the excretion of sweat on a certain skin surface, whereas the whole body sweat rate covers the total loss of sweat from the body. Changes of the sweat rate can (i) result from either physiological and/or pathophysiological changes in the body, referred to as non-iatrogenic; or (ii) be induced in the body, for example, by pharmacological therapy, referred to as iatrogenic. The sweat rate may be increased above the physiological need in regard to maintaining thermoregulation, named hyperhidrosis, or decreased, named hypohydrosis[54]. When there is no sweat excretion at all, it is called anhidrosis. Derived from common medical terminology, changes in the sweat rate are classified as focal if a limited area on the body surface is affected or generalized if the whole-body surface is presumed to be affected. Changes in the sweat rate are categorized to be primary when originating within the functional chain of the sweat gland or secondary when induced by external variables affecting the sweat gland chain [Table 2][55].
Summary of the terminology of clinical sweat assessment
Umbrella term | Specification | Description |
Sweat rate | Local | Sweat collected from a specific body surface over a specified time (indicated in g·h-1·m-2) |
Whole body sweat loss | Total amount of water lost due to sweating over a specified time | |
Causes of sweat rate changes | Iatrogenic | Sweat rate impacted through medical treatment or intervention |
Non-iatrogenic | Changes of the sweat rate that are not directly caused by medical treatment | |
Amount of sweat | Hyperhidrosis | Increased above the physiological need regarding the maintenance of the thermoregulation of the body |
Hypohidrosis | Decreased under the physiological need regarding the maintenance of the thermoregulation of the body | |
Anhidrosis | No sweating | |
Changes in the amount of sweat rate | Focal | Specific locus on the body surface is affected |
Generalized | The whole-body surface is presumed to be affected | |
Primary | Caused within the functional chain of the sweat gland | |
Secondary | Due to external variables of the chain of the sweat gland |
Clinical sweat monitoring
Both the local and the whole body sweat loss assessments are not continuously feasible and need lab infrastructure. Wearable sweat analysis to assess hydration during exertion or in hot environments is convenient and affordable[37]. These wearable sweat sensors enable sweat rate monitoring and can additionally assess changes in electrolyte concentration such as sodium, chloride, and additional biomarkers of interest[46,56]. By being coupled to smartphones, wearable sweat sensors provide a straightforward opportunity to continuously assess the local sweat rate. This, in turn, makes it possible to extrapolate the whole body sweat loss[56]. Furthermore, learning algorithms enable direct data interpretation and use within predictive models to establish preventive measures or to adapt therapies.
Sweat in clinical medicine
Hyperhidrosis
Primary hyperhidrosis is an idiopathic condition that occurs in 4.8% of the U.S. population. The lead symptom is excessive sweating. The most affected regions are the plantar, palmar, and axillary regions of the body[57,58]. While not primarily being life-threatening, primary hyperhidrosis directly affects social life. More severe causes of hyperhidrosis can be assessed during clinical anamnesis and examination. One of the more prevalent symptoms of hyperhidrosis is night sweats, which is assessed by inquiring the patients if night sweats have been observed. The current clinical definition of night sweats is when pajamas had to be changed during the night as being soaked up wet. Due to the binary nature of the question, only the affirmative answer leads to documentation and, therefore, implementation into the treatment plan. In many instances, however, night sweats are not further investigated. Increased night sweats may have several underlying reasons, such as (i) elevated environmental conditions (temperature/relative humidity); (ii) infectious diseases such as viral infections with Influenza or COVID-19; and (iii) bacterial infections such as pneumonia and even tuberculosis. Additionally, night sweats may appear in cases of (iv) autoimmune diseases or (v) cancer. Also, (vi) hormone changes, such as those seen in hyperthyroidism; (vii) genetical changes affecting the sweat gland function (e.g., in cystic fibrosis); (viii) brain infarction; or (ix) pharmacological treatments with amiodarone or hormone substitution, may lead to hyperhidrosis.
Hypohydrosis
Hypohydrosis can be categorized into exogenous, dermatological, and neurological causes[59]. Systemic neurohormonal inhibition of sweating or damage to skin and sweat glands can result from exogenous reasons. Congenital disorders lead to dermatological disorders, and neurological pathologies can be caused by autonomous dysfunction.
While hyperhidrosis can be an indicator of serious health deterioration, hypohydrosis is less often clinically significant. However, hypohydrosis can be an indicator of peripheral polyneuropathy such as in Diabetes Mellitus. Hypohydrosis can be assessed using one of the few established clinical sweat tests, namely the thermoregulatory sweat test (TST)[60]. For the TST, an indicator powder that changes color upon contact with sweat is applied to the skin. The person undergoing examination is subsequently exposed to environmental heat that usually leads to increased sweating. This process helps identify hypohydrotic skin areas.
CONCLUSION
Sweating is a common symptom in clinical medicine beyond sports science. Up to date, the absolute quantification of the sweat rate is challenging as the gold standard analysis by gravimetrical analysis is neither continuous nor feasible outside of a lab setting. These barriers are the main factors why sweat analysis has not been implemented in clinical medicine yet. Novel wearable sweat analyzing biosensors enable us to easily and continuously monitor the sweat rate independently of specialized laboratories. With the emergence of these novel biosensing devices, the sweat rate is accessible for structured clinical investigation and can serve as a novel digital biomarker. Importantly, cyber security, bioethical, and policy considerations need to be addressed for successful clinical implementation[61,62]. Clinical investigations are needed to demonstrate the additional clinical value of wearable sweat rate analysis for all stakeholders in healthcare.
DECLARATIONS
Acknowledgments
Brasier N would like to acknowledge the MedLab Fellowship from ETH Zurich, Switzerland, and an Early-career Fellowship from Collegium Helveticum, Zurich, Switzerland.
Authors’ contributions
Made substantial contributions to the conception and design of the Commentary: Brasier N, Niederberger C, Salvatore GA
Availability of data and materials
Not applicable.
Financial support and sponsorship
None.
Conflicts of interest
All authors declared that there are no conflicts of interest.
Ethical approval and consent to participate
Not applicable.
Consent for publication
Not applicable.
Copyright
© The Author(s) 2024.
REFERENCES
1. Cheshire WP, van Gerpen JA, Sejvar JJ. Sudor anglicus: an epidemic targeting the autonomic nervous system. Clin Auton Res 2020;30:317-23.
3. Brasier N, Eckstein J. Sweat as a source of next-generation digital biomarkers. Digit Biomark 2019;3:155-65.
4. Niederberger C, Vermeersch A, Davidhi F, et al. Wearable sweat analysis to determine biological age. Trends Biotechnol 2023;41:1113-6.
5. Brasier N, Ates HC, Sempionatto JR, et al. A three-level model for therapeutic drug monitoring of antimicrobials at the site of infection. Lancet Infect Dis 2023;23:e445-53.
6. Saga K. Structure and function of human sweat glands studied with histochemistry and cytochemistry. Prog Histochem Cytochem 2002;37:323-86.
7. Groscurth P. Anatomy of sweat glands. In: Kreyden RBOP, Burg G, editors. Hyperhidrosis and botulinum toxin in dermatology. Basel: KARGER; 2002. Available from: https://karger.com/books/book/2404/chapter-abstract/5731870/Anatomy-of-Sweat-Glands?redirectedFrom=fulltext. [Last accessed on 26 Dec 2023].
8. Sato K. The physiology, pharmacology, and biochemistry of the eccrine sweat gland. In: Reviews of physiology, biochemistry and pharmacology, Volume 79. Berlin, Heidelberg: Springer; 1977. pp. 51-131.
9. Sato K, Leidal R, Sato F. Morphology and development of an apoeccrine sweat gland in human axillae. Am J Physiol 1987;252:R166-80.
10. Goldsmith L. Biochemistry and physiology of the skin. Available from: https://www.semanticscholar.org/paper/Biochemistry-and-physiology-of-the-skin-Goldsmith/34a4d8b4a8433196783aac530084013e5aa531a7. [Last accessed on 26 Dec 2023]
11. Baker LB. Physiology of sweat gland function: the roles of sweating and sweat composition in human health. Temperature 2019;6:211-59.
12. Wenger CB. Heat of evaporation of sweat: thermodynamic considerations. J Appl Physiol 1972;32:456-9.
13. Harker M. Psychological sweating: a systematic review focused on aetiology and cutaneous response. Skin Pharmacol Physiol 2013;26:92-100.
14. Ando H, Noguchi R. Dependence of palmar sweating response and central nervous system activity on the frequency of whole-body vibration. Scand J Work Environ Health 2003;29:216-9.
16. Simmers P, Li SK, Kasting G, Heikenfeld J. Prolonged and localized sweat stimulation by iontophoretic delivery of the slowly-metabolized cholinergic agent carbachol. J Dermatol Sci 2018;89:40-51.
18. Nadel ER, Mitchell JW, Saltin B, Stolwijk JA. Peripheral modifications to the central drive for sweating. J Appl Physiol 1971;31:828-33.
19. Nadel ER, Bullard RW, Stolwijk JA. Importance of skin temperature in the regulation of sweating. J Appl Physiol 1971;31:80-7.
20. Nadel ER, Pandolf KB, Roberts MF, Stolwijk JA. Mechanisms of thermal acclimation to exercise and heat. J Appl Physiol 1974;37:515-20.
21. Havenith G, Fogarty A, Bartlett R, Smith CJ, Ventenat V. Male and female upper body sweat distribution during running measured with technical absorbents. Eur J Appl Physiol 2008;104:245-55.
22. Coull NA, West AM, Hodder SG, Wheeler P, Havenith G. Body mapping of regional sweat distribution in young and older males. Eur J Appl Physiol 2021;121:109-25.
23. Shapiro Y, Pandolf KB, Goldman RF. Predicting sweat loss response to exercise, environment and clothing. Eur J Appl Physiol Occup Physiol 1982;48:83-96.
24. Armstrong LE. Heat acclimatization. In: Fahey TD, editor. Encyclopedia of sports medicine and science. 1998. Available from: https://www.sportsci.org/encyc/. [Last accessed on 27 Dec 2023].
25. Pandolf K, Sawka M, Gonzalez R. Human performance physiology and environmental medicine at terrestrial extremes. 1988. Available from: https://api.semanticscholar.org/CorpusID:126430352. [Last accessed on 5 Jul 2023].
26. Institute of Medicine (US) Committee on Military Nutrition Research. Nutritional needs in hot environments: applications for military personnel in field operations. Washington (DC): National Academies Press (US); 1993. Available from: https://www.ncbi.nlm.nih.gov/books/NBK236233/. [Last accessed on 26 Dec 2023].
27. Emrich HM, Stoll E, Friolet B, Colombo JP, Richterich R, Rossi E. Sweat composition in relation to rate of sweating in patients with cystic fibrosis of the pancreas. Pediatr Res 1968;2:464-78.
28. Lezana JL, Vargas MH, Karam-Bechara J, Aldana RS, Furuya MEY. Sweat conductivity and chloride titration for cystic fibrosis diagnosis in 3834 subjects. J Cyst Fibros 2003;2:1-7.
30. Choi J, Ghaffari R, Baker LB, Rogers JA. Skin-interfaced systems for sweat collection and analytics. Sci Adv 2018;4:eaar3921.
31. Liu Y, Pharr M, Salvatore GA. Lab-on-skin: a review of flexible and stretchable electronics for wearable health monitoring. ACS Nano 2017;11:9614-35.
32. Legner C, Kalwa U, Patel V, Chesmore A, Pandey S. Sweat sensing in the smart wearables era: towards integrative, multifunctional and body-compliant perspiration analysis. Sens Actuator A Phys 2019;296:200-21.
33. Gao F, Liu C, Zhang L, et al. Wearable and flexible electrochemical sensors for sweat analysis: a review. Microsyst Nanoeng 2023;9:1.
34. Nyein HYY, Bariya M, Tran B, et al. A wearable patch for continuous analysis of thermoregulatory sweat at rest. Nat Commun 2021;12:1823.
35. Koh A, Kang D, Xue Y, et al. A soft, wearable microfluidic device for the capture, storage, and colorimetric sensing of sweat. Sci Transl Med 2016;8:366ra165.
36. Matzeu G, Fay C, Vaillant A, Coyle S, Diamond D. A wearable device for monitoring sweat rates via image analysis. IEEE Trans Biomed Eng 2016;63:1672-80.
37. Ghaffari R, Aranyosi AJ, Lee SP, Model JB, Baker LB. The Gx sweat patch for personalized hydration management. Nat Rev Bioeng 2023;1:5-7.
38. Nyein HYY, Tai LC, Ngo QP, et al. A wearable microfluidic sensing patch for dynamic sweat secretion analysis. ACS Sens 2018;3:944-52.
39. Choi DH, Gonzales M, Kitchen GB, Phan DT, Searson PC. A capacitive sweat rate sensor for continuous and real-time monitoring of sweat loss. ACS Sens 2020;5:3821-6.
40. Kim SB, Lee K, Raj MS, et al. Soft, skin-interfaced microfluidic systems with wireless, battery-free electronics for digital, real-time tracking of sweat loss and electrolyte composition. Small 2018;14:1802876.
41. Yuan Z, Hou L, Bariya M, et al. A multi-modal sweat sensing patch for cross-verification of sweat rate, total ionic charge, and Na+ concentration. Lab Chip 2019;19:3179-89.
42. Kwon K, Kim JU, Deng Y, et al. An on-skin platform for wireless monitoring of flow rate, cumulative loss and temperature of sweat in real time. Nat Electron 2021;4:302-12.
43. Liu S, Yang DS, Wang S, et al. Soft, environmentally degradable microfluidic devices for measurement of sweat rate and total sweat loss and for colorimetric analysis of sweat biomarkers. EcoMat 2023;5:e12270.
44. Emaminejad S, Gao W, Wu E, et al. Autonomous sweat extraction and analysis applied to cystic fibrosis and glucose monitoring using a fully integrated wearable platform. Proc Natl Acad Sci U S A 2017;114:4625-30.
45. Kim J, Jeerapan I, Imani S, et al. Noninvasive alcohol monitoring using a wearable tattoo-based iontophoretic-biosensing system. ACS Sens 2016;1:1011-9.
46. Tu J, Min J, Song Y, et al. A wireless patch for the monitoring of C-reactive protein in sweat. Nat Biomed Eng 2023;7:1293-306.
47. Ye C, Wang M, Min J, et al. A wearable aptamer nanobiosensor for non-invasive female hormone monitoring. Nat Nanotechnol 2023.
48. Lee H, Song C, Hong YS, et al. Wearable/disposable sweat-based glucose monitoring device with multistage transdermal drug delivery module. Sci Adv 2017;3:e1601314.
49. Drexelius A, Fehr D, Vescoli V, Heikenfeld J, Bonmarin M. A simple non-contact optical method to quantify in-vivo sweat gland activity and pulsation. IEEE Trans Biomed Eng 2022;69:2638-45.
50. Hao Q, Ren XR, Chen Y, et al. A sweat-responsive covalent organic framework film for material-based liveness detection and sweat pore analysis. Nat Commun 2023;14:578.
51. Wesson DR, Ling W. The Clinical Opiate Withdrawal Scale (COWS). J Psychoactive Drugs 2003;35:253-9.
52. Morris NB, Cramer MN, Hodder SG, Havenith G, Jay O. A comparison between the technical absorbent and ventilated capsule methods for measuring local sweat rate. J Appl Physiol 2013;114:816-23.
53. Barnes KA, Anderson ML, Stofan JR, et al. Normative data for sweating rate, sweat sodium concentration, and sweat sodium loss in athletes: an update and analysis by sport. J Sports Sci 2019;37:2356-66.
54. Smith CC, Pariser D. Primary focal hyperhidrosis. Available from: https://www.uptodate.com/contents/primary-focal-hyperhidrosis. [Last accessed on 26 Dec 2023].
55. Smetana GW. Evaluation of the patient with night sweats or generalized hyperhidrosis. Available from: https://www.uptodate.com/contents/evaluation-of-the-patient-with-night-sweats-or-generalized-hyperhidrosis. [Last accessed on 26 Dec 2023].
56. Baker LB, Seib MS, Barnes KA, et al. Skin-interfaced microfluidic system with machine learning-enabled image processing of sweat biomarkers in remote settings. Adv Mater Technol 2022;7:2200249.
57. Doolittle J, Walker P, Mills T, Thurston J. Hyperhidrosis: an update on prevalence and severity in the United States. Arch Dermatol Res 2016;308:743-9.
58. Glaser DA, Hebert A, Pieretti L, Pariser D. Understanding patient experience with hyperhidrosis: a national survey of 1,985 patients. J Drugs Dermatol 2018;17:392-6.
60. Illigens BMW, Gibbons CH. Sweat testing to evaluate autonomic function. Clin Auton Res 2009;19:79-87.
61. Perakslis ED, Ranney ML, Goldsack JC. Characterizing cyber harms from digital health. Nat Med 2023;29:528-31.
Cite This Article
Export citation file: BibTeX | RIS
OAE Style
Brasier N, Niederberger C, Salvatore GA. The sweat rate as a digital biomarker in clinical medicine beyond sports science. Soft Sci 2024;4:6. http://dx.doi.org/10.20517/ss.2023.39
AMA Style
Brasier N, Niederberger C, Salvatore GA. The sweat rate as a digital biomarker in clinical medicine beyond sports science. Soft Science. 2024; 4(1): 6. http://dx.doi.org/10.20517/ss.2023.39
Chicago/Turabian Style
Brasier, Noé, Carmela Niederberger, Giovanni Antonio Salvatore. 2024. "The sweat rate as a digital biomarker in clinical medicine beyond sports science" Soft Science. 4, no.1: 6. http://dx.doi.org/10.20517/ss.2023.39
ACS Style
Brasier, N.; Niederberger C.; Salvatore GA. The sweat rate as a digital biomarker in clinical medicine beyond sports science. Soft. Sci. 2024, 4, 6. http://dx.doi.org/10.20517/ss.2023.39
About This Article
Special Issue
Copyright
Data & Comments
Data
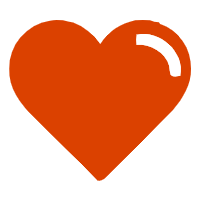

Comments
Comments must be written in English. Spam, offensive content, impersonation, and private information will not be permitted. If any comment is reported and identified as inappropriate content by OAE staff, the comment will be removed without notice. If you have any queries or need any help, please contact us at support@oaepublish.com.